Delivering on our vision for the metaverse will require us to reimagine a network infrastructure capable of supporting the computing platforms of the future. Although the metaverse is still a ways off, elements of it are already underway and we are already collaborating with telecommunications companies around the world to develop shared, open access optical fiber networks that can help support this work. By sharing costs and making network capacity available to entire market ecosystems, open networks help make abundant, affordable, high-quality internet available to more people today — and eventually, to deliver on the promise of the metaverse.
When we began thinking about surveying potential fiber routes across Democratic Republic of the Congo (DRC), we knew that paved roads (critical to laying these optical fiber cables) were in short supply, which meant we’d need a different approach to gather a rich data set to inform our construction cost estimates. In collaboration with Sofrecom and its partners Groupe CVA, and SOTEK Group, we have implemented a novel technique for optical fiber route surveys, which leverages dynamic cone penetrometers (DCP) and gamma-ray spectrometers to accelerate the survey process and improve the accuracy of cost estimates for network construction, which enables companies to quickly determine whether a new project is feasible.
The route engineering process
For most Meta fiber projects, we work with telecom industry partners to plan and deploy networks. The process starts by identifying sites Meta and our partners would like to connect, and then we use OpenStreetMap (OSM) data with our network planning tools to iterate on route options and select an optimum medium-level design (i.e., which road). After further fine-tuning designs in collaboration with project partners, we move to the final step: completing a low level design (i.e., which side of the road) using various field survey techniques meant to gather data on potential construction obstacles.
As part of the process, we also look to determine the quantity of materials required for a project as well as preferred construction methods. With this data in hand, we can then estimate both the time and cost to deploy a fiber network, both of which are key inputs to assess any project’s financial viability.
Innovation opportunity
One way of improving the accuracy of cost estimates for underground fiber networks is by classifying soil conditions. This is important, as the volume of soil to be trenched is one major cost driver and soil density is another: the harder the soil, the more effort required and the higher the cost.
One common method for gathering soil density data is with a dynamic cone penetrometer (DCP). This device estimates soil density (measured in megapascals) by correlating the force required to drive a steel rod into the ground with the rod depth achieved by that force. By repeatedly striking the rod down to the desired depth (e.g., two meters), a soil density profile from the surface to final depth can be calculated. A DCP is a relatively inexpensive instrument and it’s easy to use, but it’s not a fast process. Additionally, its measurements are highly localized, so to truly provide value, one needs to complete a lot of tests — easily dozens per kilometer — adding significant time and cost to a survey.
Another method for estimating soil density is through data gathered with a gamma-ray spectrometer. This device is fairly common in the mining industry and takes advantage of the fact that trace amounts of radioactive elements exist in many minerals. A spectrometer can detect and measure gamma radiation emissions from elements like uranium, thorium, and potassium. Such data can be analyzed and used to estimate soil density because the radiation source and radiation levels are dependent on soil composition and soil particle size. While more expensive and complex than a DCP, data collection with a spectrometer is highly automated, and a person can be quickly trained to collect data for remote post-processing and analysis by specialty geologists.
Another strength of a spectrometer is its ability to detect soil heterogeneity. It can identify where shifts in subsurface conditions occur. If emissions are similar in an area, it’s relatively safe to conclude the soil is homogeneous across the area. This is important because the spectrometer results require calibration against control data. In our case, the control data is collected via DCP.
The inspiration
Roads are critical for timely and cost-effective development of optical fiber networks. Burying fiber cables alongside roads enables easy movement of the materials, equipment, and labor required to install a network. Roads also simplify future operations, as network technicians must return to monitor and maintain the fiber for its 20-plus-year lifespan.
However, roads (and bridges) are expensive to build and maintain. This is especially true across some countries like the DRC. With a vast 2.3 million km2 geography (much of which is covered in rain forest and laced with rivers and streams) and an annual GDP per capita of about $1,000, DRC has been able to develop just 3,000 km of paved roads (similar to Luxembourg, a country 0.1 percent the size of DRC). This means fiber project logistics in DRC are complicated and costly.
Thus, when we began thinking about surveying potential fiber routes across DRC, we knew a different approach might be needed to gather a rich data set that could better inform our construction cost estimates. We went looking for an innovative and disruptive route survey technique and found it in a solution proposed by Sofrecom and its partners Groupe CVA and SOTEK Group.
Using a DCP in conjunction with a spectrometer mounted to a high-clearance 4×4 vehicle, we created a high-speed soil density classification process:
- First, a SOTEK team operating a spectrometer gathered gamma emissions, then transmitted the data to CVA in France for post-processing (spectrometer data was combined with geological data from other sources) and expert analysis.
- Preliminary results were returned to the field along with specific locations for a second SOTEK team to gather DCP measurements, which were then handed back to CVA to finalize the soil density analysis.
In this way, the number of DCP tests for the survey were minimized with a relatively small collection of DCP test results used to calibrate a vast amount of spectrometer data. In parallel with DCP measurements, an application running on a mobile device with GPS was used to capture traditional survey data points like construction obstacles, along with environmentally and culturally sensitive areas. With this process, we were able to create a detailed geographical information system map combining typical survey data with detailed soil condition information, all without extending the survey timeline.
One limitation of a spectrometer is that it cannot be used to capture data below paved surfaces, as the materials in asphalt and concrete include some of the same radioactive elements found in soils, thus any results would be highly skewed. However, with so few paved roads in DRC, we were able to turn the road conditions to our advantage.
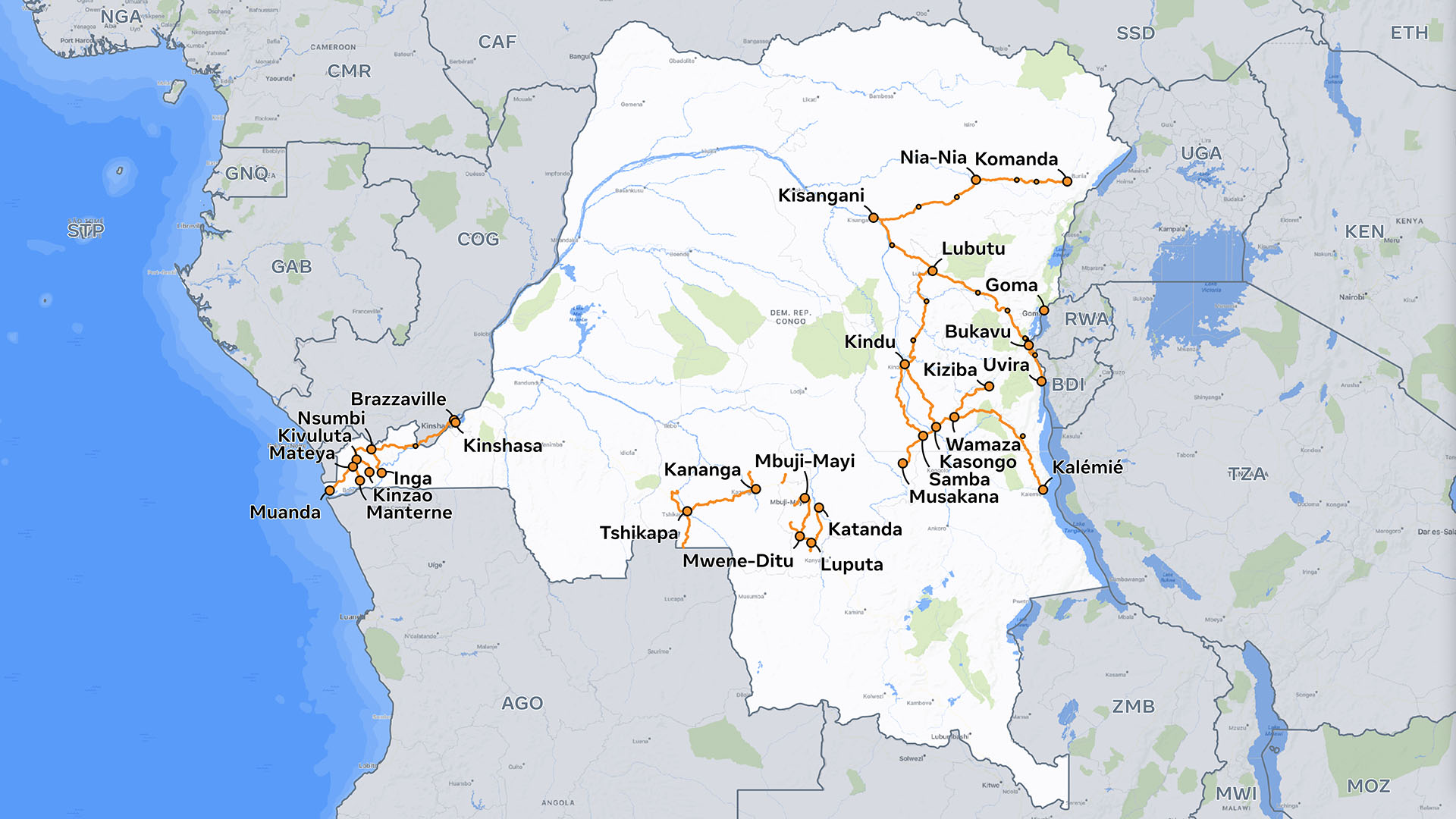
Cost risk management
Economics are often a barrier to connectivity, even more so in emerging markets, where project costs are often similar to those in developed markets. Additionally, the short-term returns are often reduced when compared with developed markets, due to factors like low internet penetration and income. We hope methods like those described above reduce project risk around the world with solid ground truth data, helping more projects achieve their initial business case hurdles and removing some of the unknowns.
What’s next?
Using this technique, we have completed surveying more than 5,000 km of potential fiber routes across DRC, but it’s possible to improve upon this method. Other technologies like hyperspectral imaging and ground penetrating radar also hold promise as data-gathering techniques. Of course, we are a leader in computer vision and machine learning, fields that are very useful for processing this type of survey. Our capabilities in these areas can be applied to these types of data sets to help future route surveys by:
- further lowering costs;
- increasing process velocity;
- increasing fidelity and accuracy; and
- enabling in-country partners to do more of this technical work locally and helping them develop their national telecoms ecosystems in the process.
Over the last decade, we have invested billions of dollars alongside telecom industry partners to improve connectivity around the world. These open, collaborative efforts include internet exchanges and carrier neutral colocation to extend the benefits of our foundational infrastructure investments like fiber and subsea cables. We’ve seen firsthand how industry-wide collaboration can improve and expand global connectivity. As we help build the foundation for the metaverse, we will continue to shepherd projects that will help equip people everywhere to thrive in this bold future.
We’d like to thank Ibrahima Ba, Fabrice Ouandji, and Clive Van Hilten for their work on this project.